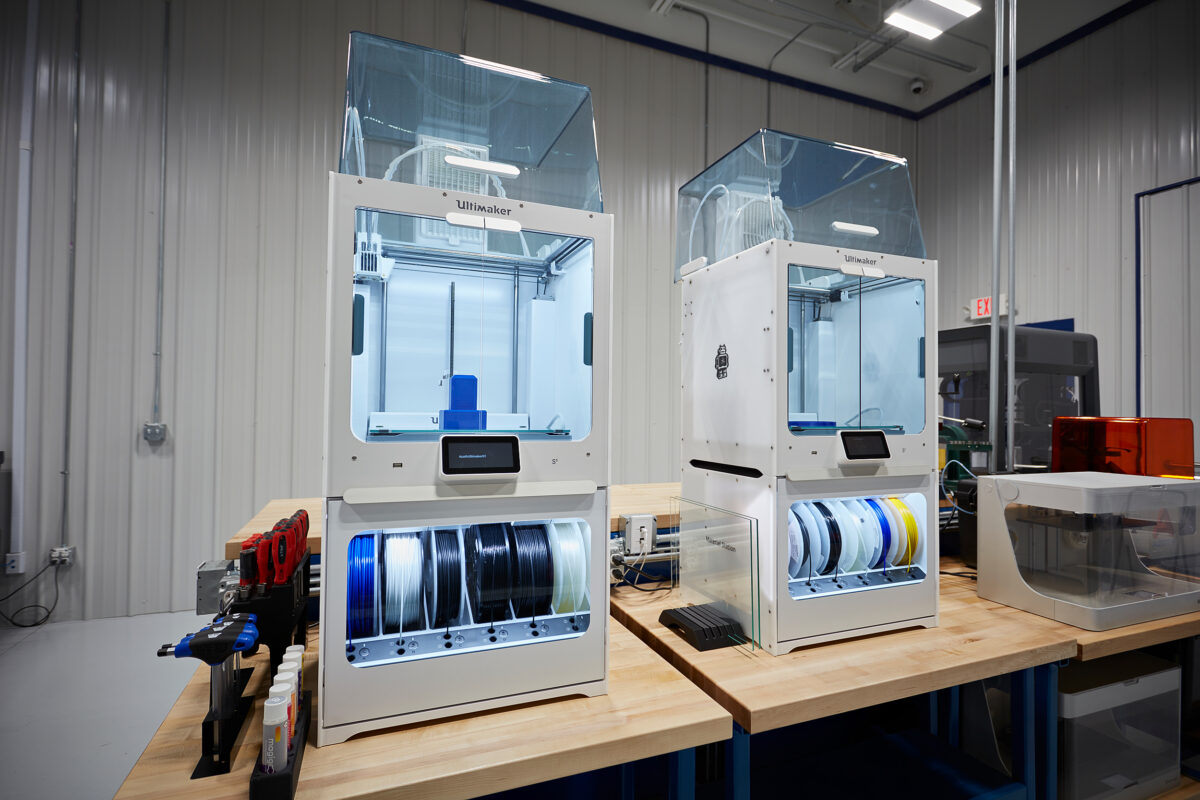
Plastic 3D Printing: Technology Overview
Filaments? Resins? Powders? The world of plastic 3D printing can indeed feel confusing and overwhelming. Within it, a multitude of technologies exists, each offering distinct advantages and unique limitations. While the foundational concept of constructing a part layer by layer remains consistent, nearly every other aspect can vary significantly. Factors such as mechanical properties, part resolution, maximum size, cost, and more are all intricately tied to the chosen printing process.
This article clarifies the subject by offering an overview of various plastic 3D printing processes, detailing common materials, and outlining their respective pros and cons.
Fused Deposition Modeling
Fused Deposition Modeling (FDM), alternatively known as Fused Filament Fabrication (FFF), is the quintessential technology that comes to mind when talking plastic 3D printing. Originating in the early 1990s, FDM 3D printing has gained widespread popularity, particularly fueled by the availability of more affordable machines, catering to both DIY enthusiasts and engineers alike.
The FDM process involves feeding thermoplastic wire, known as filament, through a heated nozzle. The nozzle then extrudes the filament onto a build plate in the X-Y plane, creating each layer of the intended part's cross-section. As the nozzle or the build plate indexes in the Z-direction, the process repeats for each subsequent layer, gradually building up the final three-dimensional object.
FDM offers a diverse selection of materials commonly used in 3D printing. This includes PLA, ABS, PETG, TPU, and nylon, which are frequently employed for various applications. For situations where enhanced strength is needed, composite filaments can be utilized. These composites blend the plastic filament with additives such as chopped glass fibers, chopped carbon fibers, or continuous Kevlar fiber, resulting in reinforced parts with superior mechanical properties.
FDM-produced parts generally offer a cost-effective solution compared to other plastic 3D printing processes and provide access to a broad spectrum of available materials. However, FDM parts may exhibit lower resolution when compared to alternative methods.
Visible layer lines are common, although surface roughness can be mitigated through post-processing techniques. Additionally, it's important to note that thermoplastic materials typically have limited heat resistance.
FDM excels in various applications including rapid and economical prototyping, concept modeling, customized consumer products, educational models, light tooling, and basic functional parts. It is particularly well-suited for limited to low-volume production scenarios.
Stereolithography and Digital Light Processing
Stereolithography (SLA) stands as one of the earliest plastic 3D printing processes, with its origins dating back to the 1980’s. SLA operates by utilizing a photocurable liquid resin and an ultraviolet laser to fabricate parts. During the build process, a build plate is submerged into a vat containing resin. The focused laser beam scans across the build plate, selectively curing the resin layer by layer. As the build plate indexes in the Z-direction, the process repeats for each subsequent layer until the desired object is formed.
Digital Light Processing (DLP) employs a similar process to SLA. However, instead of using a laser to cure the cross-section of the part, DLP utilizes a UV projector to cure the entire layer simultaneously.
Both SLA and DLP technologies provide engineered resins tailored to offer a diverse range of mechanical properties. These include resins mimicking ABS-like characteristics, flexibility, high-temperature resistance, as well as biocompatible resins and resins intended for investment casting molds.
SLA and DLP technologies offer exceptionally high part resolution and detail, making it challenging to discern layer lines with the naked eye, resulting in parts with a smooth surface finish.
However, the maximum build size is typically smaller compared to FDM and may come at a higher price point.
Additionally, SLA parts necessitate post-processing steps involving UV curing to achieve optimal material properties.
SLA and DLP excel in various applications, including jewelry, dental models, prototyping for consumer electronics, miniatures, figurines, and small medical devices. Their high-resolution capabilities and smooth surface finish make them particularly well-suited for producing intricate and detailed objects in these fields.
Selective Laser Sintering and Multi Jet Fusion
Both Selective Laser Sintering (SLS) and Multi Jet Fusion (MJF) utilize plastic powders as their feedstock. In SLS, a controlled layer of powder is evenly spread across the build plate. Subsequently, a CO2 laser scans across the build plane, selectively sintering the cross-section of the part. The build plate then moves in the Z-direction, and another layer of powder is applied, repeating the process for each subsequent layer until the part is complete.
Common plastic materials used in SLS include nylon, TPU, TPE, and polypropylene. These materials can also be reinforced with additives such as glass beads to enhance mechanical properties. Typically, SLS-
printed parts have a natural color derived from the powder, often appearing white or off-white. However, if a specific color is required, parts can be dyed or painted post-printing to achieve the desired appearance.
SLS is advantageous in that it does not necessitate support materials, enabling the fabrication of complex geometries with ease. Additionally, SLS parts have high strength and durability, rendering them suitable for both functional prototypes and end-use applications. However, it's worth noting that SLS parts may incur higher costs. While SLS offers a larger build volume than SLA, it is often slightly smaller in size to FDM.
SLS stands out in various applications, including functional prototypes for engineering purposes, customized orthotics and prosthetics, aerospace components, automotive parts, and modestly functional components. Its ability to produce strong and durable parts, coupled with its capability to handle
complex geometries, makes it a preferred choice for a wide range of industries and applications requiring high-performance materials and intricate designs.
Although both MJF and SLS utilize powdered feedstock, MJF employs an inkjet printhead to selectively apply a fusing agent onto the powder bed. Subsequently, an infrared heater fuses the powder together to form the desired part. Compared to SLS, MJF boasts significantly higher print speeds and enhanced surface finish, which in turn enables higher production volumes. This makes MJF an attractive option for applications requiring higher production volumes and improved surface finish.
Filaments, Resins, Powders, and Beyond
Plastic 3D printing encompasses a diverse family of technologies, each with its own set of advantages tailored to specific applications. Considerations such as resolution needs, material characteristics, and budgetary factors all weigh heavily in determining the most suitable technology for a given project.
Not sure what process may be best for your part? Our Application Engineers are ready to review your design and give advice. Reach out to info@azoth3d.com with any questions.
When ready, submit your parts to Azoth today!